Table of Contents
Skeletal Muscle Anatomy
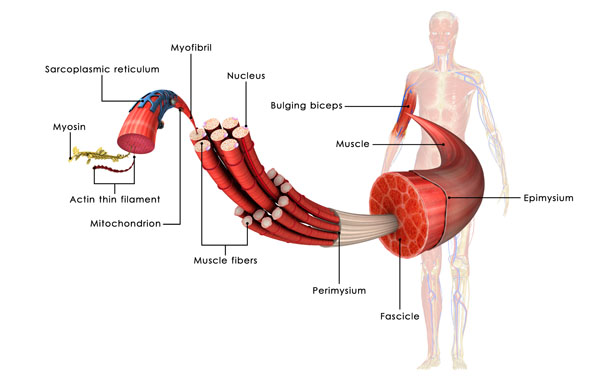
This illustration presents a detailed view of skeletal muscle anatomy and physiology, extending from the macroscopic level to the microscopic..
On the right side, we see a transparent human figure with an emphasis on the muscular system. The highlighted area focuses on the biceps brachii, a muscle of the upper arm that is responsible for flexion at the elbow and supination of the forearm.
The image zooms in on a single muscle from the biceps, illustrating its structure. The outermost layer of the muscle is the epimysium, a sheath of fibrous elastic tissue which surrounds the entire muscle. Beneath the epimysium, we find the perimysium, which encases a bundle of muscle fibers, forming a fascicle. Within each fascicle, individual muscle fibers are encased in their own protective sheath known as the endomysium.
Further magnification reveals the inner components of a single muscle fiber. Here, myofibrils, the long filaments that run parallel to the fiber, are visible. Myofibrils are composed of repeating sections called sarcomeres, which are the functional units of muscle contraction. The sarcomere is where the actual contraction process occurs, facilitated by the interaction between the thick filaments of myosin and the thin filaments of actin. The image showcases a myosin head forming a cross-bridge with actin, which is a crucial step in the contraction mechanism.
Each muscle fiber contains multiple nuclei due to their multinucleated nature, which is necessary for protein synthesis and the regulation of muscle function. The sarcoplasmic reticulum, a specialized form of endoplasmic reticulum, is also featured, which stores and releases calcium ions to trigger muscle contraction.
Lastly, mitochondria are shown, which are the powerhouses of the cell, providing the energy required for muscle contractions through the production of ATP (adenosine triphosphate). These organelles play a critical role in energy transfer during muscle activity.
This image serves as a comprehensive illustration of the hierarchical organization of muscle tissue, from its gross anatomy in the body down to the molecular mechanisms of muscle contraction.
Illustration of the Human Muscular System
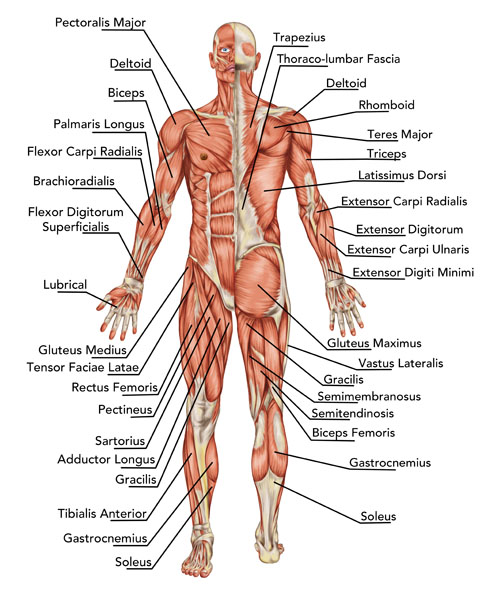
The illustration displays an anterior view of the human muscular system, labeling various muscles in the upper and lower limbs, torso, and back. At the top, we see the pectoralis major, the large chest muscle that is crucial for the movement of the shoulder joint. The deltoid muscle caps the shoulder, responsible for lifting the arm and giving the shoulder its rounded contour.
On the arm, several muscles are identified. The biceps brachii, commonly known as the biceps, is a prominent muscle on the front of the upper arm and is involved in flexing the elbow and rotating the forearm. Below the biceps, the brachioradialis and flexor carpi radialis are forearm muscles that flex the elbow and wrist, respectively. The palmaris longus, a muscle that can vary in presence among individuals, is also labeled, and it acts to flex the hand at the wrist.
On the back of the arm, we can identify the triceps brachii, the large muscle responsible for extending the elbow. Further down, the extensor muscles of the forearm are shown, including the extensor carpi radialis, which extends and abducts the wrist, and the extensor digitorum, which extends the fingers.
Moving to the lower body, the gluteus maximus, the largest and most superficial of the three gluteal muscles, is responsible for the movement of the hip and thigh. The tensor fasciae latae, a muscle of the thigh, stabilizes and steadies the hip and knee during activities like running. The quadriceps group, including the rectus femoris and the vastus lateralis, are powerful extensors of the knee joint and are critical for walking, running, jumping, and squatting.
The sartorius, the longest muscle in the human body, runs down the length of the thigh and aids in flexing, abducting, and laterally rotating the hip. The adductor longus and gracilis are part of the inner thigh muscles, which bring the thighs together. The hamstrings, including the semitendinosus and biceps femoris on the posterior thigh, are essential for knee flexion and hip extension.
Lower down, the gastrocnemius muscle forms the bulk of the calf and is involved in running, jumping, and other fast movements of the leg, while the soleus, situated beneath the gastrocnemius, plays a significant role in standing and walking.
The muscular anatomy shown here is essential for numerous movements and stability across various joints in the human body.
Muscle Architecture
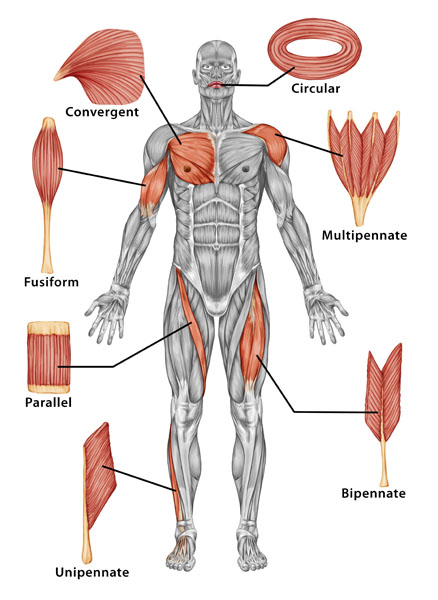
The image provides a visual guide to different types of muscle architectures in the human body, correlating muscle shapes with specific functions.
At the top, we see a circular muscle, exemplified by the orbicularis oris around the mouth, which allows the mouth to close or pucker as seen in actions like speaking and kissing.
The convergent muscle is represented by the large, fan-shaped pectoralis major muscle in the chest. This type of muscle has a broad origin and converges to a single tendon, allowing for versatile movements but not as much force.
The deltoid muscle is shown as an example of multipennate architecture, where many fibers are arranged at multiple angles around a central tendon, resembling the barbs on a feather. This configuration provides a high power output.
The biceps brachii muscle is illustrated as a fusiform muscle, which is spindle-shaped with a central belly that tapers to tendons on each end, allowing for a good balance between power and range of motion.
A parallel muscle, like the rectus abdominis in the abdomen, has fibers that run parallel to the long axis of the muscle, providing a greater range of motion but less power compared to other types.
The biceps femoris, part of the hamstrings group in the thigh, is an example of a bipennate muscle, where fibers run obliquely on both sides from a central tendon, resembling a feather. This structure allows for a greater force production but a smaller range of motion.
Lastly, the unipennate tibialis posterior muscle in the lower leg, where the fibers run obliquely from a single side of a central tendon, provides a combination of strength and stability to the foot.
Each muscle type is specialized for different functions in the body, whether it be fine control, power, or endurance, and the diversity of muscle architectures allows for the wide range of movements and activities humans can perform.
Innervation of Skeletal Muscles
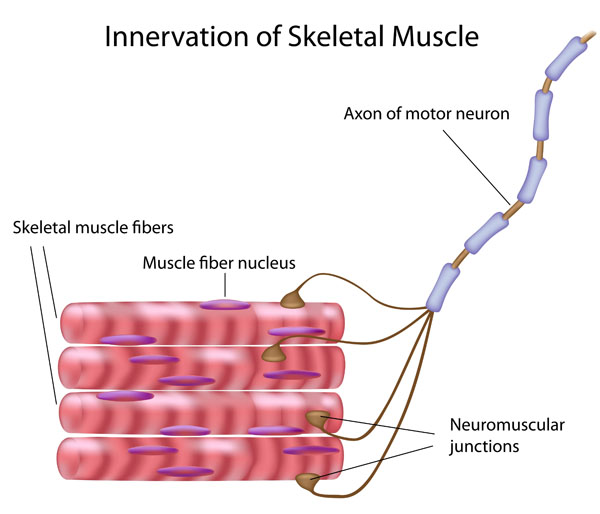
This illustration depicts the innervation of skeletal muscle, showing how motor neurons transmit signals to muscle fibers to initiate contraction.
The axon of a motor neuron is shown branching out towards individual skeletal muscle fibers. Motor neurons are the nerve cells responsible for conveying impulses from the brain or spinal cord to the muscles, thereby controlling their activity.
At the point where the motor neuron meets the muscle fiber, there is a specialized synaptic connection called the neuromuscular junction. It is at this juncture that the neuron’s electrical signal is converted into a chemical signal, which then triggers an electrical signal in the muscle fiber, causing it to contract.
The muscle fiber is a single cylindrical cell, and like other cells, it contains nuclei that house the cell’s genetic material and are involved in regulating the cell’s activities, including the production of proteins necessary for muscle contraction.
This system of motor neurons and muscle fibers is essential for all voluntary muscle movements, and the precision of this communication allows for coordinated and controlled muscle contractions.
Process of Muscle Contraction and Relaxation
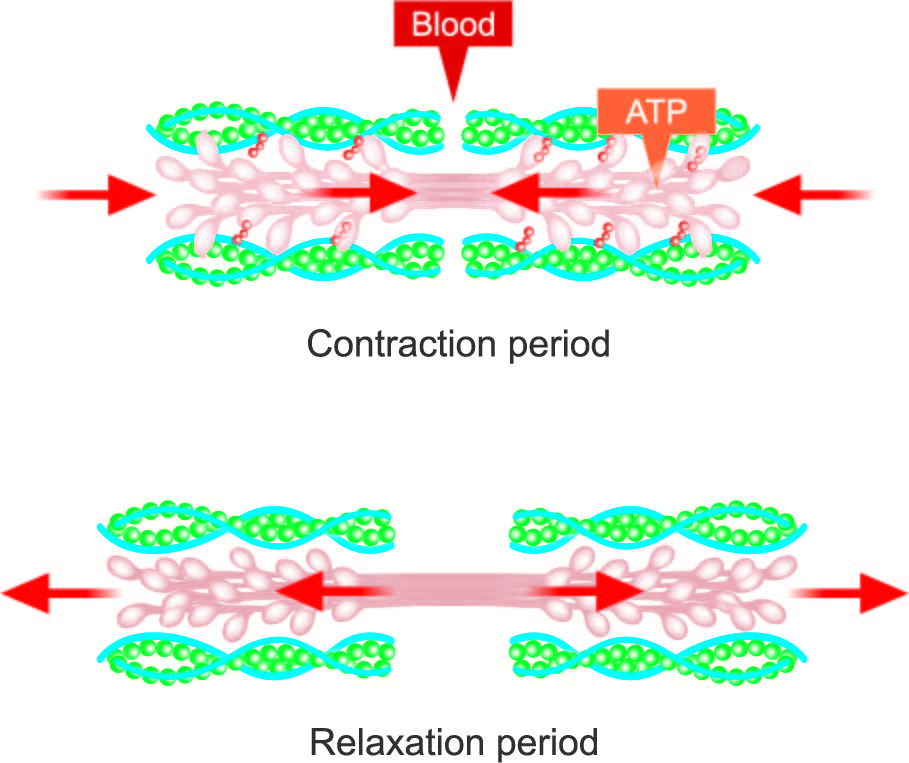
This diagram is a simplified representation of the processes of muscle contraction and relaxation, specifically at the level of the sarcomeres within muscle fibers.
In the contraction period at the top, we see the sarcomeres in a shortened state. The sarcomeres are the basic contractile units of muscle fibers, composed of overlapping thick (myosin) and thin (actin) filaments. During contraction, myosin heads bind to actin filaments and pull them closer together, a process powered by ATP (adenosine triphosphate), the energy currency of the cell. This action is depicted by the arrow labeled “ATP,” indicating the direction of energy transfer. The shortening of these sarcomeres results in the muscle fiber contracting.
The diagram also indicates that blood is involved, likely referencing the delivery of oxygen and nutrients, including ATP, to the muscle cells, which are essential for sustaining muscular contractions.
In the relaxation period at the bottom, the sarcomeres return to their elongated resting state. This occurs when the myosin heads release the actin filaments, an action that also requires ATP. The absence of a stimulus from a motor neuron and the active pumping out of calcium ions from the muscle fiber contribute to this relaxation.
The arrows on both sides represent the force generated during contraction and relaxation. During the contraction period, the arrows are directed inward, denoting the muscle shortening as the sarcomeres come closer together. During relaxation, the arrows are directed outward, illustrating the muscle returning to its original length.
Overall, this diagram encapsulates the cyclical nature of muscle contraction and relaxation, emphasizing the critical role of ATP in both processes.
Types of Muscle Tissue
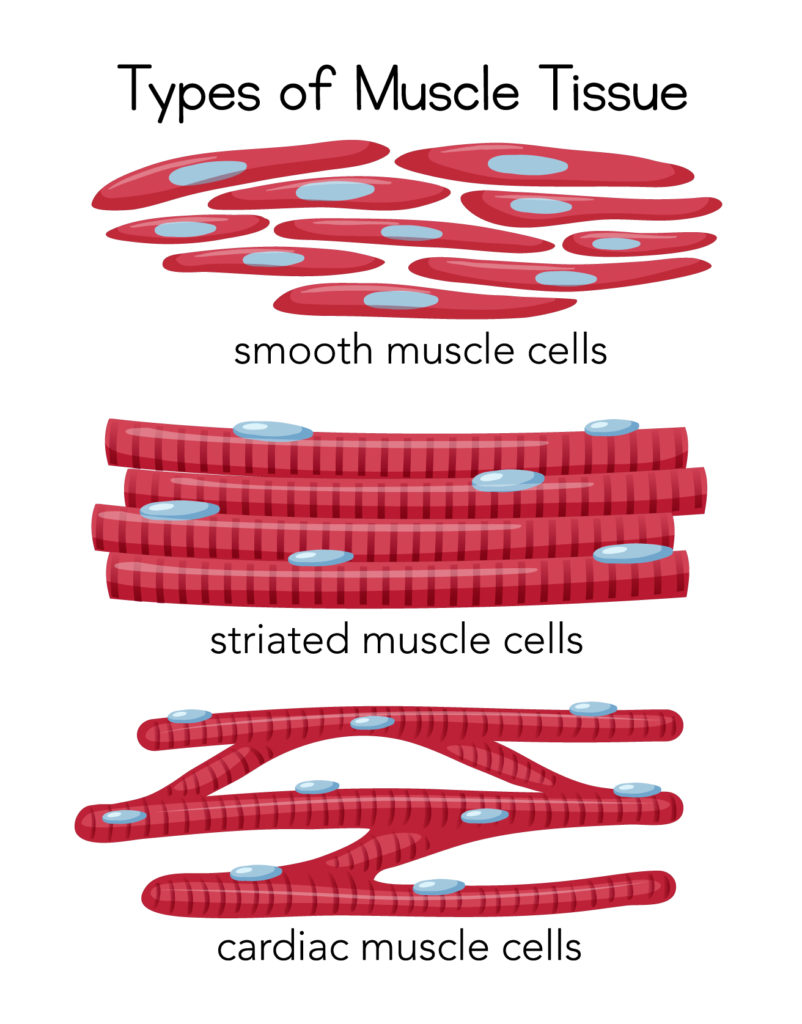
The illustration depicts the three primary types of muscle tissue found in the human body: smooth muscle cells, striated muscle cells, and cardiac muscle cells, each with distinct structures correlating to their specific functions.
At the top, we have smooth muscle cells, which are spindle-shaped with a single central nucleus. These cells are non-striated, meaning they do not have the visible banding pattern seen in other muscle types. Smooth muscle makes up the walls of internal organs such as the intestines, blood vessels, and the bladder, and its contractions are involuntary, controlled by the autonomic nervous system.
In the middle, striated muscle cells are illustrated, which are also known as skeletal muscle fibers. These cells are cylindrical, multinucleated, and display a regular pattern of light and dark bands, known as striations, which are due to the precise arrangement of myofilaments within the cells. These are the muscles that attach to bones and are under voluntary control, responsible for body movements.
At the bottom, cardiac muscle cells are shown. Cardiac muscle tissue is unique to the heart and shares some characteristics with both smooth and striated muscle. These cells are branched, striated, and typically have a single nucleus. They are connected to each other by intercalated discs, which allow for rapid transmission of electrical impulses, facilitating the heart’s rhythmic contractions. Cardiac muscle contractions are involuntary and are rhythmically initiated by the heart’s own pacemaker cells.
This image serves as a visual summary of the different muscle types, highlighting the structural differences that enable their diverse roles in the human body.
Structure and Function of a Sarcomere
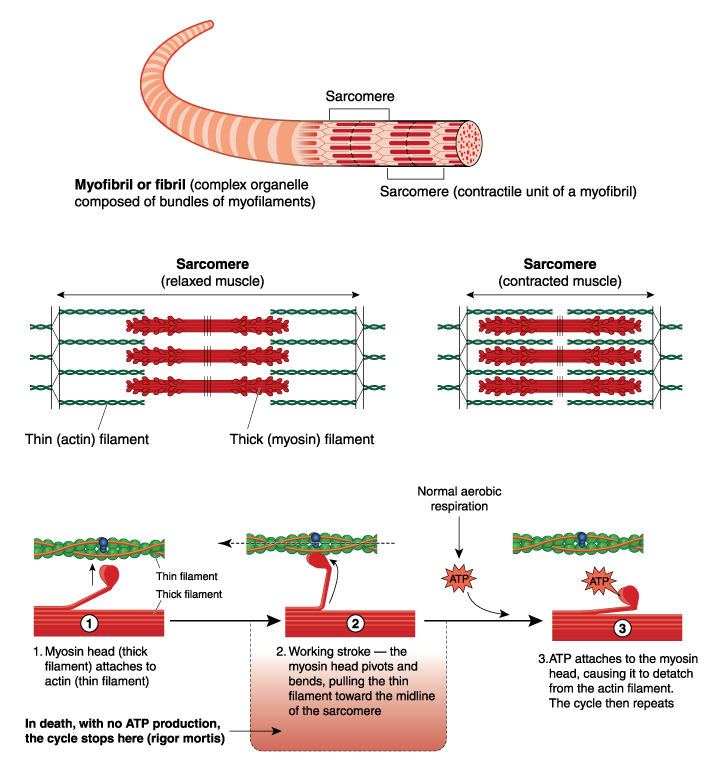
This diagram provides a detailed explanation of the structure and function of a sarcomere, the basic contractile unit of muscle fiber, and the molecular mechanism of muscle contraction.
At the top left, we see an elongated muscle fiber with a zoomed-in section showing the myofibrils, which are composed of bundles of myofilaments. These myofibrils are made of repeating units called sarcomeres, highlighted in the circular inset, which show the organized arrangement of thick and thin filaments.
The central part of the image compares a sarcomere in a relaxed state to one that is contracted. In the relaxed sarcomere, the thick (myosin) and thin (actin) filaments overlap only slightly at the ends of the sarcomere. When the muscle contracts, as seen in the right image, the thick and thin filaments slide past each other, and the sarcomere shortens, with the zones of overlap increasing and the entire length of the sarcomere decreasing. This sliding filament theory is the basis for muscle contraction.
The bottom part of the image illustrates the molecular process of muscle contraction, divided into three steps, occurring at the level of the myofilaments within the sarcomere:
- The myosin head, which is part of the thick filament, attaches to the actin filament, forming a cross-bridge.
- The myosin head pivots, pulling the thin filament toward the center of the sarcomere in a power stroke, utilizing energy released from ATP hydrolysis.
- A new ATP molecule attaches to the myosin head, causing it to detach from the actin filament and allowing the cycle to repeat.
The image also indicates that this cycle is dependent on the availability of ATP. In the absence of ATP, such as after death, the cycle stops, leading to rigor mortis, where the muscles stiffen due to the inability of the myosin heads to detach from actin.
The process shown is a vital physiological mechanism underpinning how muscles contract and produce force, enabling movement and stability in the body.
Anatomical Terms and Definitions
Term | Definition |
---|---|
Actin | Thin filaments within a sarcomere that interact with myosin to facilitate muscle contraction. |
Articular Cartilage | The smooth, white tissue that covers the ends of bones where they come together to form joints. It reduces friction in the joints and acts as a cushion between the bones. |
Biceps Brachii | A muscle of the upper arm that is responsible for flexion at the elbow and supination of the forearm. |
Blood Vessel | Tubular structures carrying blood through the tissues and organs; a vein, artery, or capillary. |
Bone | A rigid organ that constitutes part of the vertebrate skeleton, supporting and protecting various organs of the body, and providing leverage for muscle action. |
Deep Fascia | A layer of fibrous connective tissue which surrounds and separates the muscles. |
Deltoid Muscle | A large, triangular muscle covering the shoulder joint that is responsible for lifting the arm and giving the shoulder its range of motion. |
Endomysium | A fine sheath of connective tissue wrapping each individual muscle fiber. |
Epimysium | A fibrous tissue envelope that surrounds the entire muscle. |
Fascicle | A bundle of skeletal muscle fibers surrounded by perimysium, a type of connective tissue. |
Mitochondria | Organelles that act as the powerhouse of the cell, providing the energy required for muscle contractions through the production of ATP (adenosine triphosphate). |
Motor Neuron | A neuron that transmits impulses from the brain or spinal cord to a muscle or gland, initiating contraction or secretion. |
Muscle Fiber | A single muscle cell, especially one of those making up the skeletal muscles. Muscle fibers are elongated, multinucleated, and capable of contracting when stimulated. |
Myofibril | A long filamentous organelle found within muscle cells that has a banded appearance and is composed of sarcomeres, which contract the cell. |
Myosin | Thick filaments within a sarcomere that interact with actin filaments to produce muscle contraction by forming cross-bridges and pulling the actin filaments towards the center of the sarcomere. |
Neuromuscular Junction | The synapse or junction where the axon terminal of a motor neuron transmits a signal to the muscle fiber, causing muscle contraction. |
Perimysium | The connective tissue that surrounds each fascicle (bundle of muscle fibers). |
Sarcolemma | The plasma membrane surrounding a muscle fiber, which helps to conduct action potentials and maintains the ionic balances of the muscle fiber. |
Sarcomere | The basic contractile unit of muscle fiber in striated muscle, consisting of a linear array of repeating units, delineated by Z-discs, containing the thin (actin) and thick (myosin) filaments whose interaction leads to muscle contraction. |
Sarcoplasmic Reticulum | A specialized form of endoplasmic reticulum in muscle cells, storing and releasing calcium ions to trigger muscle contraction. |
Tendon | A band of fibrous connective tissue that usually connects muscle to bone and is capable of withstanding tension. |
Z-disc | A protein disc within the sarcomere of muscle fibers to which the thin filaments (actin) are attached at either end, defining the boundaries of each sarcomere. |